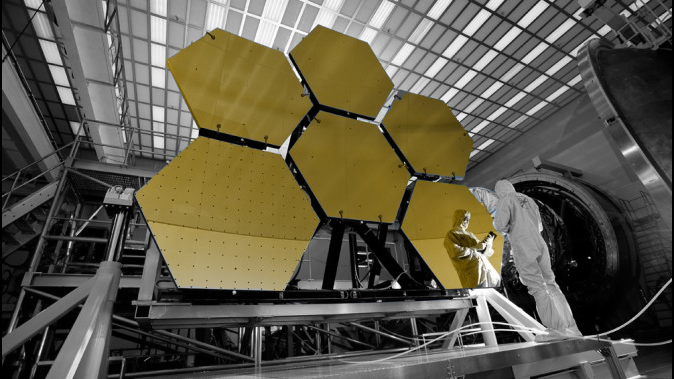
Image made through Photoshop by Palak
Part 1: Overview
If you consider yourself a part of the science community but you’ve been living under a rock for the past 5 months and don’t know JWST enough to be considered ‘Webbers’, no worries! Your chain of hysterical searches has landed you at the perfect place. The significance of Sir James E. Webb can be found here. Let’s talk science, shall we…
The James Webb Space Telescope (JWST) is the finest, most advanced space telescope recently launched into space on the 25th of December, 2021. It is meant for Infrared astronomy, which is observing/studying the universe with electromagnetic radiation in the range of 600nm (visible red) to 28,000nm or 28m (mid-infrared). This is a range rather narrower and far ‘redder’ than what Hubble can observe and does not include much of the visible spectrum but this range is just right to observe:
We want to peer deep into the emptiness to look further in space and hence backwards in time to the formation of the very first stars and galaxies. Due to the expansion of the universe, the light emitted from these ancestor stars is redshifted by the Doppler effect.
2. Obscured objects.
Visible light observations do not account for the dust clouds that generally block the object of interest. Most of the higher frequency light coming from the object gets scattered by this dust but most of the low-frequency light gets through hence observing in the ‘redder’ part of the spectrum allows one to look through the dust clouds expanding the boundaries of the research.
3. Hunt for exoplanets.
In comparison to stars which outshine their orbiting planets in the ultra-violet and visible region, exoplanets and even some objects in our solar system (objects in the Oort cloud) are very faint and cool enough to radiate heat in the infrared range.
Such visionary outcomes also demanded years, almost 30 years of hard work and resilience.
Numerous challenges, some unfortunate and some fortunate events over the years came down to the telescope finally being fully crafted in October 2021. Before counting down, with the interest of the non-webbers in mind, we look into some of the engineering marvels of this piece.
The Optics:
- It has the ‘Three Mirror Astigmat’ arrangement of optics. This system gets rid of most optical aberrations and is the best fit for capturing a wider field of view.
- Its primary mirror (the most prominent golden hexagon shape you see) is made up of 18 smaller hexagonal segments which when completely unfolded will make a mirror with a diameter of 6.5m giving us a total light-collecting area of 25 m2 (about 6.25 times the area of the Hubble Space Telescope).
Source: https://webbtelescope.org/contents/media/images/4181-Image
- Yet it is half as light as Hubble and can detect up to 100 times fainter objects.
- Fitting a non-segmented circular mirror of the same size in the rocket would’ve been impossible hence the hexagonal shaped mirrors provide the most efficient space-filling capacity while also maintaining a somewhat circular shape. Folding it like origami paper could be easily achieved in this configuration of mirrors.
- Segmenting the primary mirror created another problem. It has to unfold and perfectly align with each other with no gaps in between to form an even surface while also maintaining its curvature. Implausible as it may seem, the scientists made it possible and to such a precision that “each mirror is aligned to 1/10,000th the thickness of a human hair”, as claimed by Lee Feinberg, Webb Optical Telescope Element Manager at NASA Goddard.
To put this into perspective, 1/10,000th thickness of a hair is approximately 7nm which means the misalignment only has a margin of 50 atoms or so. That is an amazingly reflective (shiny) surface, smoother than any telescopic non-segmented mirror so far.
- Each of the 18 segments is 1.32m in diameter with its base skeleton made of beryllium being 1mm thick and a pure Gold coating on top only 700 atoms (100nm) thick. Beryllium fits the category of ‘light yet strong’ and holds its structure through drastic changes in temperatures. It has also been used in previous space missions because of this reason. A gold coating (done with Vacuum Vapor Deposition technique) enhances its ability to focus infrared light.
The Sun Shield:
Source: https://webb.nasa.gov/content/observatory/sunshield.html
- 5 sheets, each within a millimetre thick, made up of Kapton with an aluminium coating (also includes other dopped substances) take the shape of a kite with dimensions of (2212)m, as big as a tennis court.
- Since all the observations are to be done under 50K to detect the faintest infrared signals, an SPF of >1 million was needed as the side facing the sun will be at around 400K. This sun shield is designed to not only do the former but also protect the optics and the instruments from meteorite damage. The whole sun shield is divided into grids, by the method of Thermal Spot Bond to limit the spread of the tear from the meteorite.
- The layers work by successively reflecting light and heat from the sun and the internal heat of the instruments, spreading it out into space. The vacuum left between the layers acting as an additional insulator adds to the sun shield’s efficiency.
The Orbiting position:
- The observatory will be orbiting around the Lagrange Point 2 (L2) of the earth-sun system.
Source: https://i.stack.imgur.com/aM9L5.jpg
- In short Lagrange Points in a 3-Body system are points where gravitational forces between two bodies balance out such that the third body can remain in stable equilibrium at that position. The earth-sun system has 5 Lagrange points. A lot of space observatories operate at one of these Lagrange points but L2 is the most promising for JWST as it is the farthest from the sun, earth and moon or any heat sources.
- Around this position, the telescope can be conveniently placed in orbit and simultaneously face away from the sun peering into the darkness of the universe.
There are 4 main instruments onboard. The 3 near-infrared (NIR) instruments will work under 40K temperature, passively cooled by the sun shield and the Mid-infrared instrument (MIRI) will operate at an astounding temperature of <7K, for this, it has an active cooling mechanism.
- NIRCam is a camera with about 250,000 micro shutters arranged like a waffle grid. Each shutter is individually controlled and can provide simultaneous imaging of specific objects of interest. The best part about dividing the camera aperture into so many windows is that it can resolve light coming from objects appearing too close to each other (maybe 2 or 3 star systems or eclipsing planets or if the two objects are almost the same line of sight).
- NIRSpec is the near-infrared spectrograph with a resolution so high that it can make spectroscopic observations of 100 objects in an area smaller than the Hubble deep field (9sq.arcmin to be exact) at the same time, more than any instrument ever flown before.
Each detector in the NIR instruments has about 4 million pixels.
- The FGS (Fine Guidance Sensor) /NIRISS (Near-Infrared Imager And Slitless Spectrograph) is a single instrument with two parts, the FGS is the ‘fine-tuner’ for the cameras used for stable pointing for high exposure. It can detect angular movement as small as human hair at a distance of 1 kilometre. Its guiding system checks for an object’s position on the frame almost 16 times per second.
The NIRISS will be most useful in detecting exoplanet atmospheres and transits using Aperture Masking Interferometry.
- The MIRI will detect very faint sources like hardly visible comets and objects in the Kuiper belt. It is actively cooled by a ‘cryocooler’ onboard which makes use of a pulse tube precooler and then the Joule-Thomson loop cooling method to reach its final operating temperature. The instrument has a detector having 1 million pixels.
“We are set up to see and we think we might see objects after a redshift of 20 or 30”, claimed Dr John C. Mather (SmarterEveryDay, 2021)
Up to the redshift of z = 20 (180 million years cosmic time after the Big Bang), for comparison, the earliest stars are thought to have formed between z≈30 and z≈20 (100-180 million years cosmic time), the first galaxies may have formed around redshift z≈15 (about 270 million years cosmic time), and Hubble is unable to see further back than very early reionization at about z≈11.1 (galaxy GN-z11, 400 million years cosmic time).
As much of an engineering marvel as this telescope is, it not only has to survive the thrust and vibrations of its launch into space but also navigates to L2, unfolds automatically and survives and operates for 10 further years with no possibility for repair missions. On the 25th of December 2021, its fate was sealed. To know what happened after T-minus 2 months, tune in tomorrow for the next part!
What does “half as light” mean? To me, that means twice as heavy. Did you mean that, or did you mean half as heavy? What I read suggests Hubble weighed 24,000 lbs at launch, and JWST weighed 14,200 lbs. That means grammatically, I think you meant to say that JWST is half as heavy as Hubble.